The Revolutionary Field of Nanomedicine - Impact factor international journal of nanomedicine
The revolutionary field of nanomedicine , where nanotechnology meets healthcare. Learn how nanoscale materials are transforming diagnosis, treatment, and prevention of diseases - impact factor international journal of nanomedicine
SCIENCE PAPERS
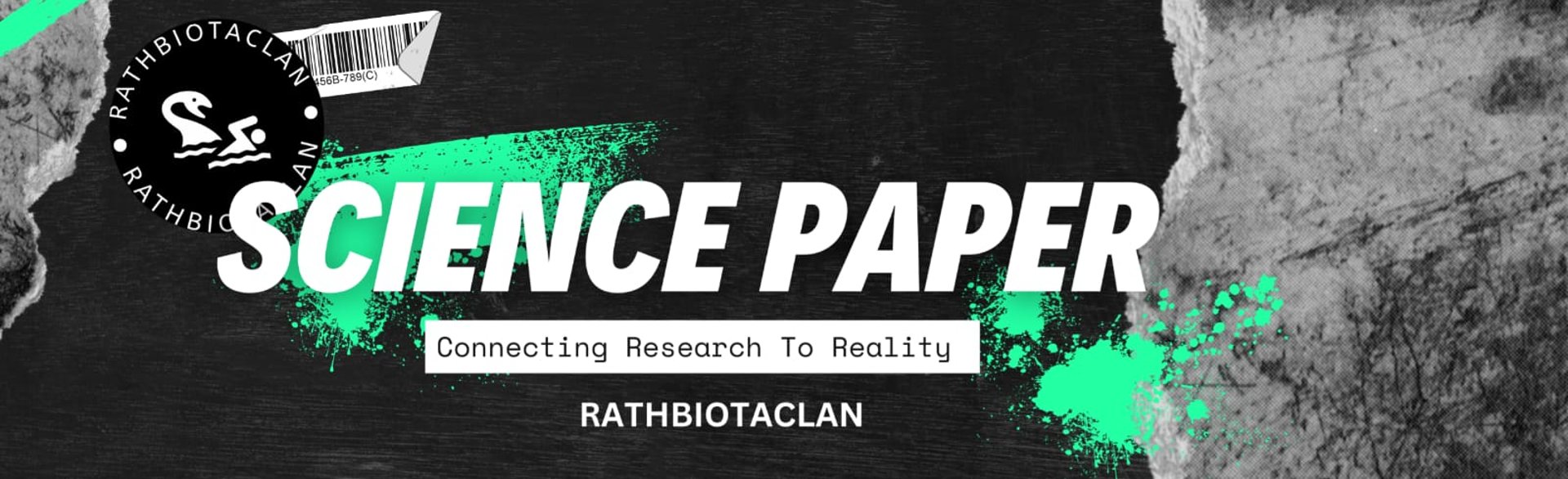
Nanomedicine
Introduction
Nanomedicine is an emerging field that applies nanotechnology to innovate in healthcare. It focuses on the use of nanoscale materialsβtypically between 1 and 100 nanometers in sizeβto interact with the human body at the molecular level. Nanomedicine holds the potential to revolutionize how we diagnose, treat, and prevent diseases.
Nanoparticles, which can be naturally occurring or human-made from materials like carbon or silver, are central to nanomedicine. These human-engineered substances possess unique properties that differ from their larger-scale counterparts, allowing for highly specific and targeted medical applications.
Impact of Nanomedicine on Healthcare
Scientists in nanomedicine are developing materials and devices that operate at the atomic or molecular scale within the body. This precise level of interaction allows for targeted treatments that can minimize side effects and improve therapeutic outcomes, offering a promising future for more personalized and effective healthcare solutions.
Revolution of Drug delivery by Nanomedicine
The rise of nanotechnology has also led to significant advancements in drug delivery systems. Nanomedicine has facilitated the creation of novel drug-delivery vehicles that can overcome the limitations of traditional methods. These advanced systems improve the pharmacokinetics and pharmacodynamics of drugs, enhancing their stability, bioavailability, and therapeutic index while minimizing off-target effects.
Nanomaterials (NMs) are rapidly transforming the landscape of technology and medicine due to their extraordinary and versatile properties. These materials, which range in size from 1 to 100 nanometers, exhibit chemical, physical, and biological characteristics that differ significantly from those of their larger counterparts. This distinctiveness has opened up new avenues in various scientific disciplines, particularly in the burgeoning field of Nanomedicineβa domain where the frontiers of nanotechnology intersect with biology, chemistry, and engineering to revolutionize healthcare.
Nanomedicine represents a shift in how we approach medical treatment and diagnostics. Its versatility allows for the development of highly specialized nanodevices and materials that can be tailored to address specific clinical needs. This field is not limited to a single application but spans a broad range of medical advancements. Researchers are exploring Nanomedicine's potential in creating more effective vaccines, novel drug delivery systems, and advanced diagnostic tools that can detect diseases at their earliest stages.
One of the most exciting areas of Nanomedicine is its potential to transform cancer treatment. Traditional cancer therapies often struggle with precision, leading to damage to healthy tissues and severe side effects. Nanomedicine offers a solution by enabling the development of targeted drug delivery systems that can home in on cancer cells with high specificity. For example, researchers are currently investigating the use of gold nanoparticles conjugated with specific antibodies to target and destroy cancer cells while sparing healthy ones. This approach could significantly reduce the side effects of chemotherapy and improve patient outcomes.
Another promising avenue in Nanomedicine is the development of nanoscale sensors and diagnostic tools. These devices can detect biomarkers at extremely low concentrations, enabling early diagnosis of diseases such as Alzheimer's, Parkinson's, and various forms of cancer. A recent breakthrough involves the use of carbon nanotubes in creating highly sensitive biosensors that can detect disease markers in blood samples long before symptoms appear. Such early detection is crucial in improving the chances of successful treatment.
The COVID-19 pandemic underscored the critical role Nanomedicine can play in addressing global health crises. The rapid development of mRNA vaccines by Pfizer-BioNTech and Moderna, which utilized lipid nanoparticles for efficient delivery of the genetic material, demonstrated how Nanomedicine could be leveraged for rapid and effective response to emerging infectious diseases. This success has spurred further research into nanotechnology-based vaccines for other viral diseases, such as HIV and influenza.
Beyond infectious diseases and cancer, Nanomedicine is also making strides in regenerative medicine. Researchers are developing nanomaterials that can promote tissue regeneration and repair, offering new hope for patients with severe injuries or degenerative diseases. For instance, scientists are exploring the use of nanofibers as scaffolds for tissue engineering, which could potentially lead to the development of artificial organs. These nanofibers can mimic the extracellular matrix of tissues, providing a framework for cells to grow and form new tissue.
In the realm of antibiotic resistance, which poses a significant threat to global health, Nanomedicine is offering innovative solutions. Nanoparticles are being engineered to deliver antibiotics directly to bacterial cells, bypassing mechanisms that bacteria use to resist conventional treatments. Additionally, researchers are investigating the use of metallic nanoparticles, such as silver and copper, which have inherent antimicrobial properties, to develop new types of antibiotics that bacteria cannot easily resist.
While the potential of Nanomedicine is vast, it is not without challenges. One of the most significant hurdles is understanding how nanomaterials interact with biological systems at the molecular level. This knowledge is crucial for ensuring the safety and efficacy of nanomedicine-based treatments. To address these challenges, interdisciplinary collaborations between biologists, chemists, engineers, and clinicians are essential. These collaborations are leading to the development of advanced computational models that can predict the behavior of nanomaterials in biological environments, thereby accelerating the translation of Nanomedicine from the laboratory to the clinic.
Ethical and regulatory considerations also play a critical role in the future of Nanomedicine. As these technologies move closer to clinical application, ensuring that they meet safety standards and are accessible to all will be paramount. Ongoing discussions around the ethical implications of nanotechnology in medicine, including issues of equity, consent, and long-term effects, are essential in shaping the responsible development of this field.
Nanomedicine is not just a promise for the future; it is an evolving reality that is already making a significant impact on healthcare. With continued research and development, it holds the potential to revolutionize how we diagnose, treat, and prevent diseases, ultimately improving the quality of life for people around the world. As we stand on the brink of this new era in medicine, the integration of nanotechnology into clinical practice will likely become as commonplace as the stethoscopeβan indispensable tool in the physician's arsenal.
Importance of Nanomedicine for targeted therapy
Nanomedicine enables targeted therapy by functionalizing nanoparticles with specific ligands or antibodies that bind selectively to diseased cells or tissues. This targeted approach not only increases drug concentration at the site of action but also reduces systemic toxicity, resulting in more effective treatments. Additionally, these nanocarriers can be designed to respond to specific stimuli, such as pH or temperature, ensuring that the drug is released only where it is needed.
Nanomedicine-based imaging methods, including MRI, PET, and fluorescence imaging, enhance sensitivity and specificity, enabling early disease detection, accurate diagnosis, and real-time monitoring of treatment responses.
By integrating nanomedicine with diagnostics, researchers are creating multifunctional platforms that can simultaneously diagnose and treat diseases. These platforms allow for the customization of treatments based on an individual's specific molecular profile, leading to better therapeutic outcomes and fewer side effects.
Finally, the concept of theranostics, which combines diagnostics and therapeutics into a single entity, is another application of nanomedicine.
This approach involves the integration of imaging agents, therapeutic agents, and targeting molecules within a single nanoparticle, enabling clinicians to visualize disease sites and administer treatment simultaneously, offering a comprehensive and personalized treatment strategy.
Nanotechnology in medicine
Nanotechnology in medicine, often referred to as nanomedicine, involves the application of
nanotechnologyβmanipulating matter on an atomic or molecular scaleβto develop new tools, treatments, and techniques that can significantly enhance healthcare.
Nanomedicine utilizes materials and devices that are incredibly small, typically between 1 and 100 nanometers in size, to interact with biological systems at the cellular and molecular levels.
This nanoscale interaction allows for highly targeted and precise approaches to diagnosing, treating, and even preventing diseases, offering potential advancements over traditional medical practices.
For Case, in drug delivery, nanotechnology enables the development of nanoparticles that can carry drugs directly to specific cells or tissues in the body, minimising side effects and improving the effectiveness of treatments. These nanoparticles can be designed to release their therapeutic payload only when they reach the targeted site, reducing damage to healthy cells and enhancing the overall treatment outcome.
Moreover, nanotechnology is being used to create advanced diagnostic tools. Nanoparticles with unique optical or magnetic properties are being developed to improve imaging techniques, allowing for earlier detection of diseases like cancer. These enhanced imaging methods can provide clearer and more accurate pictures of whatβs happening inside the body, leading to better diagnosis and monitoring of diseases.
Another exciting application of nanotechnology in medicine is in the field of personalized medicine. By analyzing a patientβs genetic makeup, nanotechnology can help create tailored treatments that are specific to an individualβs needs, leading to more effective therapies with fewer side effects.
What Role Does Nanotechnology Play in Precision Medicine ?
Nanotechnology plays a crucial role in precision medicine by enabling highly personalized and targeted treatment approaches. In precision medicine, treatments are tailored to the individual characteristics of each patient, often based on genetic, environmental, and lifestyle factors. Nanotechnology enhances this personalization by improving the detection, diagnosis, and treatment of diseases at the molecular level.
Nanoparticles can be engineered to target specific cells or tissues, such as cancer cells, delivering drugs directly to the affected area while minimizing damage to healthy tissues. This targeted delivery reduces side effects and increases the effectiveness of treatments. Additionally, nanotechnology is used in the development of advanced diagnostic tools that can detect diseases at earlier stages, allowing for timely intervention.
For example, quantum dots and gold nanoparticles are used in molecular imaging and diagnostics, providing highly sensitive detection of biomarkers that indicate the presence of a disease. This level of precision is essential for developing personalized treatment plans that are more effective and less toxic.
Nanotechnology enhances precision medicine by enabling more accurate diagnostics, targeted drug delivery, and personalized treatment strategies, ultimately improving patient outcomes.
The Potential of Nanomedicine in Healthcare
Nanomedicine has the potential to revolutionise healthcare by offering unprecedented precision and efficacy in diagnosis, treatment, and prevention. By utilizing materials and devices at the nanoscale (typically less than 100 nanometers), nanomedicine can interact with biological systems at the molecular level, leading to innovative solutions that were previously unattainable.
1. Targeted Drug Delivery:
One of the most significant potentials of nanomedicine lies in targeted drug delivery. Traditional drug therapies often suffer from systemic side effects and poor targeting of disease sites. Nanoparticles can be engineered to deliver drugs directly to specific cells or tissues, such as cancer cells, minimizing side effects and enhancing therapeutic efficacy. For example, nanoparticles can be designed to release their drug payload only in the acidic environment of a tumor, sparing healthy tissues from exposure.
2. Early and Accurate Diagnostics:
Nanomedicine also holds promise for improving diagnostic accuracy and speed. Nanoparticles can be engineered to bind to specific biomarkers associated with diseases like cancer, heart disease, or infectious diseases. This allows for the detection of diseases at much earlier stages than current methods, improving the chances of successful treatment. Additionally, the integration of nanotechnology in imaging techniques, such as MRI or CT scans, enhances the clarity and detail of images, leading to more precise diagnoses.
3. Regenerative Medicine and Tissue Engineering:
Nanotechnology can be used to create scaffolds for tissue regeneration, mimicking the extracellular matrix and supporting the growth of new tissues or organs. This is particularly promising for treating injuries or degenerative diseases where tissue repair is needed. Nanomaterials can also be used to deliver growth factors or genes to stimulate the body's own repair mechanisms.
4. Personalised Medicine:
Nanomedicine paves the way for personalised medicine, where treatments are tailored to the individual's genetic profile and specific disease characteristics. By delivering drugs or therapeutic agents in a controlled manner, nanomedicine can ensure that the right amount of medication reaches the right place at the right time, improving the effectiveness of treatment and reducing side effects.
5. Overcoming Biological Barriers:
Nanoparticles can be designed to cross biological barriers that are difficult for conventional drugs, such as the blood-brain barrier. This opens up new possibilities for treating neurological disorders, such as Alzheimer's disease or brain tumors, where delivering drugs to the brain has been a significant challenge.
The potential of nanomedicine in healthcare is vast. It offers the ability to diagnose diseases earlier, treat them more effectively, and even prevent them from occurring in the first place. As research continues to advance, nanomedicine could lead to significant breakthroughs in how we understand, manage, and treat a wide range of medical conditions, ultimately improving patient outcomes and quality of life.
Engage with Us:
Stay tuned for more captivating insights and News. Visit our Blogs and Follow Us on social media to never miss an update. Together, let's unravel the mysteries of the natural world.
Molecular Mechanism of Targeted Drug Delivery with Nanomedicine
Targeted drug delivery using nanomedicine represents a significant advancement in the field of pharmacology, offering precision treatment by delivering therapeutic agents directly to diseased tissues or cells while sparing healthy ones. This approach is particularly beneficial in treating conditions like cancer, where conventional therapies often cause extensive damage to normal cells.
Components of Targeted Drug Delivery
The molecular mechanism of targeted drug delivery with nanomedicine can be broken down into three primary stages: targeting and binding, cellular uptake via endocytosis, and controlled drug release.
1. Targeting and Binding:
The first step in targeted drug delivery involves the design of nanocarriersβtiny particles ranging from 1 to 100 nanometers in size. These nanocarriers are engineered to recognize and bind to specific receptors on the surface of target cells. This is achieved through receptor-ligand interactions. Receptors are proteins found on the surface of cells, often overexpressed in diseased tissues like tumors. Ligands are molecules that can specifically bind to these receptors.
Nanocarriers are functionalized with ligands that have a high affinity for these receptors. For example, certain cancer cells overexpress receptors like HER2, which can be targeted by nanocarriers coated with ligands such as monoclonal antibodies or small peptides. This specificity ensures that the nanocarriers preferentially accumulate at the disease site, thereby increasing the drug concentration at the target location and reducing systemic exposure.
2. Cellular Uptake via Endocytosis:
Upon binding to the target cell, the nanocarrier is internalized through a process called endocytosis. Endocytosis involves the engulfing of the nanocarrier by the cell membrane, forming an intracellular vesicle. This vesicle typically fuses with endosomes or lysosomes within the cell.
The intracellular environment plays a crucial role in drug release. For instance, lysosomes possess a low pH and contain various hydrolytic enzymes, making them an ideal site for the activation of certain drug release mechanisms. This process ensures that the drug is delivered precisely to the intended intracellular compartment, further enhancing the therapeutic effect while minimizing side effects.
3. Controlled Drug Release:
Once inside the target cell, the nanocarrier must release its payload in a controlled manner. Several strategies are employed to achieve this:
- Linker Cleavage: Many nanocarriers utilize cleavable linkers to attach the drug to the carrier. These linkers are designed to break under specific conditions, such as the acidic pH of lysosomes or the presence of specific enzymes. For example, ester and hydrazone linkers are sensitive to pH changes, while disulfide linkers are cleaved in the presence of reducing agents like glutathione, which is abundant in the cytoplasm.
- Non-Covalent Interactions: In some cases, drugs are encapsulated within nanocarriers rather than covalently bound. The drug is released through diffusion or degradation of the carrier, with the release rate controlled by factors such as the particle size, shape, and surface chemistry of the nanocarrier. These parameters are carefully optimized to ensure that the drug is released at a therapeutic rate within the target cells.
- External Triggers: Some nanocarriers are designed to respond to external stimuli such as light, temperature, or magnetic fields. For example, thermosensitive liposomes can release their contents when exposed to hyperthermia, making them useful in combination with thermal therapies for cancer.
Advantages and Challenges
The precision of targeted drug delivery using nanomedicine offers several advantages over traditional therapies. It increases the efficacy of treatment by concentrating the drug at the disease site, reduces the required dosage, and minimises systemic side effects. This is particularly important in the treatment of cancer, where the toxicity of chemotherapeutic agents can severely impact the patient's quality of life.
However, there are challenges to this approach. Designing nanocarriers that can effectively target specific cells and release drugs in a controlled manner requires a deep understanding of both the disease biology and the pharmacokinetics of the drug. Additionally, the potential for immune system recognition and clearance of nanocarriers, as well as the complexity of large-scale manufacturing, are hurdles that need to be addressed.
The molecular mechanisms underpinning targeted drug delivery in nanomedicine are complex but hold great promise for improving the specificity and efficacy of treatments. As research progresses, this approach is likely to become a cornerstone in the management of various diseases, particularly those where current treatments are inadequate or excessively toxic.
Nanomedicine Revolutionizes Cellular-Level Drug Delivery
Nanomedicine is transforming the landscape of drug delivery at the cellular level, offering unprecedented precision and control in administering therapeutic agents. The advent of nanoparticle-based drug delivery systems represents a significant leap forward, addressing many limitations of traditional therapies, such as poor targeting, off-target effects, and the need for high dosages.
Precision Targeting with Nanoparticles___
At the core of nanomedicineβs impact is the use of nanoparticlesβtiny particles typically ranging from 1 to 100 nanometers in sizeβthat can be engineered to carry drugs directly to diseased cells or tissues. These nanoparticles are often functionalized with ligands, antibodies, or peptides that specifically bind to receptors overexpressed on the surface of target cells, such as cancer cells. This targeted approach ensures that the drug is delivered precisely where it is needed, minimizing exposure to healthy tissues and reducing the potential for side effects.
For example, in cancer therapy, nanoparticles can be designed to recognize and bind to receptors like HER2 on the surface of breast cancer cells. Once bound, the nanoparticles can be internalized by the cell, delivering their therapeutic payload directly to the intracellular environment. This targeted delivery not only increases the concentration of the drug at the site of action but also allows for lower dosages, reducing the overall toxicity of the treatment.
Enhanced Drug Delivery Through Nanoparticle Design___
Nanoparticles possess unique physical and chemical properties that make them highly effective for drug delivery. One of the most important characteristics is their high surface-area-to-volume ratio, which enhances their interaction with biological molecules and cellular membranes. This property allows nanoparticles to cross biological barriers, such as the cell membrane, more efficiently than larger particles, facilitating better drug uptake and distribution within the cell.
The versatility of nanoparticles is another key advantage. They can be engineered in various shapes, sizes, and compositions, allowing for customization based on the specific therapeutic needs. For instance, nanoparticles can be constructed from materials like lipids (liposomes), polymers, or inorganic substances (such as gold or silica). Each type of nanoparticle offers distinct advantages, such as biocompatibility, stability, and the ability to carry large drug payloads.
Moreover, nanoparticles can be designed with multiple functionalities. They can carry not only therapeutic agents but also imaging molecules for diagnostic purposes, targeting sequences for specific tissues, and coatings that enhance biocompatibility or prevent immune system recognition. This multifunctionality makes nanoparticles powerful tools in the development of personalized medicine, where treatments are tailored to the specific characteristics of a patientβs disease.
Overcoming Biological Barriers___
One of the significant challenges in drug delivery is overcoming biological barriers, such as the blood-brain barrier (BBB), which protects the central nervous system but also prevents most drugs from entering the brain. Nanoparticles have shown promise in crossing the BBB, making them valuable in the treatment of neurological conditions like brain cancer, Alzheimerβs disease, and other neurodegenerative disorders. By attaching targeting molecules that recognize specific receptors on the BBB, nanoparticles can be guided across this barrier, delivering drugs directly to the brain.
In addition to the BBB, nanoparticles can be designed to bypass or exploit other biological barriers within the body. For example, nanoparticles can be engineered to evade the immune systemβs detection, allowing them to circulate longer in the bloodstream and reach their target more effectively. This capability is particularly important for treating conditions where rapid clearance of the drug would otherwise reduce its efficacy.
Intracellular Drug Delivery___
Nanoparticles are not only effective at targeting specific cells but also at delivering drugs to specific locations within the cell. For many drugs, the site of action is within the cytoplasm or the nucleus, where they can interact with cellular machinery to exert their therapeutic effects. However, getting drugs to these intracellular locations can be challenging due to cellular defense mechanisms, such as efflux pumps, that expel foreign substances from the cell.
Nanoparticles can help overcome these challenges by acting as intracellular depots, releasing their drug payload gradually within the cell. This controlled release ensures a sustained therapeutic effect, maintaining higher concentrations of the drug where it is needed most. For instance, nanoparticles can be designed to release their contents in response to specific triggers within the cell, such as changes in pH or the presence of certain enzymes. This level of control enhances the drugβs efficacy and reduces the likelihood of resistance developing.
Nanomedicine is revolutionizing cellular-level drug delivery by leveraging the unique properties of nanoparticles to achieve precise, controlled, and targeted therapy. By overcoming traditional barriers to drug delivery and providing new ways to interact with cells and tissues, nanomedicine offers the potential for more effective treatments with fewer side effects. As research in this field continues to advance, nanoparticles are likely to play an increasingly central role in the development of personalised and highly effective therapeutic strategies for a wide range of diseases.
Advancement And Innovation Nanotechnology-Based Therapeutics
Multifunctional Nanocarriers
Multifunctional nanocarriers are nanoparticles that can carry multiple therapeutic agents, such as drugs, genes, and proteins, and target specific cells and tissues. This allows for combination therapy and personalized medicine. These nanocarriers can be designed to release their payload in response to specific stimuli, such as pH or temperature changes, ensuring optimal drug delivery and minimizing off-target effects. Multifunctional nanocarriers have shown promise in treating various diseases, including cancer, genetic disorders, and infectious diseases. Researchers are exploring various materials and designs to optimize their performance and safety.
Nanoparticle-Based Immunotherapies
Nanoparticle-based immunotherapies involve using nanoparticles to stimulate immune responses against cancer cells and infectious diseases. Nanoparticles can be engineered to present antigens to immune cells, activating an immune response. They can also be used to deliver immunotherapeutic agents, such as checkpoint inhibitors, directly to immune cells. This approach has shown promise in treating various types of cancer and infectious diseases. Researchers are exploring different nanoparticle designs and materials to optimize their performance and safety.
Nanoparticle drug delivery systems represent a cutting-edge approach to administering therapeutic agents in a more precise and controlled manner. These systems utilize nanoparticles, which are designed to target specific areas in the body, ensuring that the medication is released exactly where itβs needed. This precision not only helps in minimizing side effects but also reduces the overall dosage and frequency with which the drug needs to be taken. Over recent years, nanoparticles have gained significant interest due to their potential to revolutionize drug delivery.
One of the reasons nanoparticles are so effective in drug delivery is their unique chemical and physical properties. Unlike their larger-scale counterparts, nanoparticles have a high surface-area-to-volume ratio, which allows for better interaction with biomolecules. This interaction facilitates the crossing of cell membranes, making the delivery process more efficient. Additionally, the large surface area of nanoparticles offers ample space for drugs and other small molecules, such as ligands or antibodies, to attach. This feature is crucial for targeting specific cells or tissues and for controlling the release of the therapeutic agents over time.
The appeal of nanoparticles extends across various scientific disciplines due to their versatility. Researchers can engineer nanoparticles to incorporate a wide range of properties that would be difficult to combine in a single device using traditional methods. These properties include the ability to attach biologically active molecules, targeting sequences, imaging agents like fluorescent markers, and biocompatible coatings. Moreover, scientists can manipulate the size, shape, and structure of the nanoparticle core, providing an additional level of control to tailor the particles for specific functions.
This blog delves into the application of nanoparticles in cellular and intracellular drug delivery, examining different types of nanoparticles, such as ceramics and liposomes. It also covers the current methods used to develop inorganic nanoparticles. The discussion further explores the pharmacokinetic parameters, which include the movement of drugs within the body, and the targeting strategies that enhance the effectiveness of these nanoparticles. Given the challenges posed by the blood-brain barrier in the central nervous system, the blog highlights examples of how nanoparticles are being used to treat neurological conditions, including brain cancer, neurovascular disorders, and neurodegenerative diseases.
In recent years, medical treatments have become increasingly personalized, with therapies tailored to address specific diseases and individual patient needs. Many drugs target cells and tissues directly, and ideally, they should be delivered to these specific sites within the cell for maximum effectiveness. Achieving selective subcellular delivery can greatly enhance therapeutic outcomes. For instance, delivering drugs to the cytosolβthe liquid found inside cellsβcan be particularly beneficial for medications that are otherwise expelled from the cell by efflux transporters like multi-drug resistance proteins and P-glycoproteins. These transporters often reduce the drugβs concentration within the cell, diminishing its therapeutic impact. By using intracellular nanoparticles, which can act as drug depots inside the cell, itβs possible to maintain higher concentrations of the drug where it is most needed, thereby improving treatment efficacy.
Targeted Gene Delivery
Targeted gene delivery involves using nanoparticles to deliver genes to specific cells and tissues, allowing for targeted modification of gene expression. This has implications for treating genetic disorders and diseases. Nanoparticles can be designed to target specific cells or tissues, ensuring that the gene is delivered to the intended site. Researchers are exploring various materials and designs to optimize the efficiency and safety of gene delivery.
Smart Nanomaterials
Smart nanomaterials are materials that can respond to environmental stimuli, such as pH, temperature, or light, releasing therapeutic agents in a controlled manner. This ensures optimal drug delivery and minimises off-target effects. Smart nanomaterials can be designed to release their payload in response to specific stimuli, such as changes in pH or temperature, ensuring optimal drug delivery. Researchers are exploring various materials and designs to optimize their performance and safety.
Nanoparticle-Based Combination Therapies
Nanoparticle-based combination therapies involve using nanoparticles to deliver multiple therapeutic agents simultaneously, enhancing treatment outcomes. Researchers are exploring the synergistic effects of combining nanoparticles with other therapeutic modalities, such as radiation or phototherapy. This approach has shown enhanced treatment outcomes in preclinical studies, particularly in cancer treatment.
Personalized Nanomedicine
Personalized nanomedicine involves designing nanoparticles that target specific molecular profiles or biomarkers, tailoring treatment to individual patients' needs. Advances in nanotechnology enable the development of personalized nanomedicines, improving treatment efficacy and reducing side effects. Researchers are exploring various materials and designs to optimize personalised nanomedicine.
Nanoparticle-Based Diagnostic Tools
Nanoparticle-based diagnostic tools involve using nanoparticles to detect and diagnose diseases at an early stage. Nanoparticles can be designed to target specific biomarkers or molecular profiles, enabling early disease detection and monitoring. Researchers are exploring various materials and designs to optimize nanoparticle-based diagnostic tools.
Biodegradable and Biocompatible Nanomaterials
Biodegradable and biocompatible nanomaterials are essential for translating nanotechnology-based therapeutics to the clinic. Researchers are developing biodegradable and biocompatible nanomaterials, reducing toxicity and improving safety profiles. This is crucial for ensuring the safe use of nanoparticles in humans.
Nanoparticle-Based Delivery of CRISPR/Cas9
Nanoparticle-based delivery of CRISPR/Cas9 involves using nanoparticles to deliver CRISPR/Cas9 gene editing tools, enabling precise genome modification. This has implications for treating genetic diseases. Researchers are exploring various materials and designs to optimize nanoparticle-based delivery of CRISPR/Cas9.
Artificial Intelligence in Nanomedicine
Artificial intelligence (AI) is being integrated into nanomedicine, enabling optimized nanoparticle design, personalized medicine, and predictive analytics. AI can analyze large datasets to identify optimal nanoparticle designs and predict treatment outcomes. This fusion of technologies has the potential to revolutionize healthcare.
How Does Nanomedicine Address Complex Diseases ?
1. Targeted Drug Delivery
Traditional drugs often affect both diseased and healthy cells, leading to side effects. Nanomedicine improves this by using tiny particles, called nanoparticles, to deliver drugs precisely to the target cells. These nanoparticles can be designed to recognize and attach to specific types of cells, like cancer cells. Imagine a missile programmed to hit only the intended target and avoid everything else. This approach helps in:
- Reducing Side Effects: By concentrating the drug at the disease site, the impact on healthy tissues is minimized.
- Increasing Effectiveness: The drug can be delivered in higher concentrations directly to where it's needed, making it more effective.
2. Enhanced Imaging and Diagnostics
Early and accurate detection of diseases is crucial for effective treatment. Nanomedicine enhances imaging techniques used to spot diseases:
- Contrast Agents:
Nanoparticles can be engineered to improve the visibility of tissues and organs during imaging scans like MRI, CT, or PET. This helps doctors see smaller tumors or changes that might be missed with traditional imaging.
- Biomarker Detection:
Nanoparticles can also be used to detect specific molecules associated with diseases. For example, they can bind to cancer biomarkers, making them easier to identify in blood tests.
3. Therapeutic Interventions
Nanomedicine allows for the development of new therapies:
- Gene Therapy: Nanoparticles can be used to deliver genetic material into cells, correcting genetic defects or modifying gene expression. This can potentially treat genetic disorders at the molecular level.
- Localised Treatment: For diseases like cancer, nanoparticles can release drugs directly within the tumor, avoiding the need for systemic administration and reducing overall drug toxicity.
4. Personalised Medicine
Everyone's disease and response to treatment can be different. Nanomedicine helps tailor treatments to individual patients:
- Customized Therapies:
By understanding the specific genetic and molecular profile of a patientβs disease, treatments can be tailored to target the unique characteristics of that patientβs condition.
- Adaptive Treatment:
Nanomedicine tools can help monitor how well a treatment is working, allowing for adjustments to be made in real-time.
5.Real-Time Monitoring
Monitoring disease progression and treatment response can be challenging:
- Nanosensors:
Tiny sensors made from nanomaterials can be implanted in the body to continuously measure biomarkers or changes in the disease state. For example, they can track glucose levels in diabetic patients or detect tumor markers in cancer patients.
- Immediate Feedback:
This real-time data helps doctors make informed decisions and adjust treatments promptly.
Nanomedicine uses advanced technology to manipulate matter at an extremely small scale, allowing for more precise diagnostics, targeted therapies, and personalised treatment plans. This approach holds great promise for improving the management of complex and challenging diseases.
Nanomedicine in Cancer Treatment: A New Frontier
For years, translating successful cancer treatments from the lab to clinical practice has been challenging. Issues like toxicity, delivery difficulties, stability, targeting precision, and resistance have made it hard to apply promising in vitro and in vivo results to actual patients. To overcome these barriers, nanoparticles have emerged as a powerful tool in drug delivery systems, offering new hope in cancer therapy.
Nanoparticles have been designed in various forms to improve the effectiveness of cancer treatments. For example, while immune checkpoint blockades (ICBs) have shown promise as anti-cancer drugs, their severe side effects have limited their use. By integrating ICBs with nanotechnology, researchers have developed more effective delivery methods, combining the benefits of both approaches to combat tumours more safely and efficiently.
Moreover, combining immunogenic cell death (ICD) inducers with immune checkpoint inhibitors has shown promise in preventing cancer spread and recurrence. Nanoparticles play a crucial role in this process by turning "cold" tumours, which are typically resistant to treatment, into "hot" tumours, which are more responsive to therapies.
Nanomedicine offers many advantages in cancer treatment, including targeted drug delivery, reduced side effects, and improved bioavailability. By focusing on delivering drugs directly to cancer cells, nanomedicine minimises harm to healthy tissues and enhances the overall effectiveness of treatments. As this field continues to evolve, it promises to revolutionise how we approach cancer therapy, offering new avenues for treatment and hope for millions affected by this devastating disease.
Nanomedicine and Gene Therapy: Enhancing Cancer Treatment
Gene therapy has emerged as a promising approach to treat various genetic disorders, including cancer. However, the challenge has always been the efficient delivery of genetic material to the targeted site without causing harm to healthy cells. This is where nanomedicine comes into play, offering innovative solutions for overcoming these barriers.
The development of nanoparticles is a crucial step in achieving targeted drug and gene delivery. These specialised nanoparticles are designed with unique properties that allow them to deliver genetic material precisely to the desired site within the body. Their ability to target specific cells reduces the risk of affecting non-cancerous tissues, thereby minimising side effects.
However, the process is not without challenges. One significant hurdle in nanoparticle-mediated gene delivery is ensuring consistency and reproducibility in their production. Additionally, there's the concern of potential toxicity to host cells. Overcoming these obstacles is essential for the successful application of nanoparticles in gene therapy.
A strategic approach to nanoparticle fabrication and a thorough understanding of their biological behaviour can significantly improve the success rate of nano drug and gene delivery systems. By refining these techniques, researchers can enhance the efficiency of gene therapy, making it a more viable and effective option for treating cancer.
At Base, while nanoparticle-mediated gene therapy holds great promise, it requires careful consideration of the challenges involved. With continued research and development, nanotechnology could revolutionise cancer treatment, offering new hope to patients worldwide.
Introduction to Nanomedicine and Immunotherapy
Cancer is one of the most complex and challenging diseases to treat. Among the emerging therapies, nanomedicine and immunotherapy stand out for their potential to revolutionise cancer treatment. Nanomedicine uses nanoscale materials, typically 1-100 nanometers, to deliver drugs directly to tumours, aiming to maximise efficacy while minimising side effects. Immunotherapy, which leverages the body's immune system to target and destroy cancer cells, has shown remarkable success in achieving long-term remission, especially in advanced cancers. However, each approach has its limitations, and combining them could enhance therapeutic outcomes.
The Promise of Immunotherapy
Immunotherapy has transformed cancer treatment by enabling the immune system to recognize and attack tumours. Techniques such as immune checkpoint inhibitors and adoptive T cell therapy have demonstrated significant success, especially in cases where conventional therapies have failed. However, immunotherapy is effective in only a subset of patients, and many tumours remain resistant. This has driven research into combining immunotherapy with other modalities, such as nanomedicine, to enhance its efficacy.
Combining Nanomedicine and Immunotherapy
Combining nanomedicine with immunotherapy, a strategy known as nano-immunotherapy, aims to overcome the limitations of each approach. Nanomedicine can enhance the delivery of immunotherapeutic agents to the tumour site or modify the tumour microenvironment to make it more susceptible to immune attack. This combination has the potential to convert "cold" tumours, which do not respond to immunotherapy, into "hot" tumours that are more easily targeted by the immune system.
Strategies in Nano-Immunotherapy
Nano-immunotherapy can be implemented through several approaches, each targeting different aspects of cancer biology:
1. Targeting Cancer Cells: Nanoparticles can be designed to induce immunogenic cell death in cancer cells, which not only kills the tumour cells but also releases tumour antigens and danger signals. These signals attract immune cells, enhancing the immune response against the cancer.
2. Modulating the Tumour Microenvironment: Tumours often create an immunosuppressive microenvironment that protects them from immune attack. Nanomedicines can be used to alter this environment, inhibiting suppressive cells and molecules, and boosting the activity of immune cells such as cytotoxic T cells.
3. Enhancing the Peripheral Immune System: Nanomedicines can also target the peripheral immune system, such as lymph nodes, to enhance antigen presentation and boost the production of immune cells that can attack the tumour.
Preclinical and Clinical Development of Nano-Immunotherapy
Although most nano-immunotherapy approaches are still in the preclinical stage, early clinical trials have shown promising results. These trials suggest that combining nanomedicine with immunotherapy could significantly improve patient outcomes, particularly in cancers that are resistant to traditional treatments. However, translating these findings into clinical practice requires overcoming challenges such as identifying biomarkers that can predict patient responses.
The Role of Biomarkers in Nano-Immunotherapy
Biomarkers are crucial for the development of personalised medicine, including nano-immunotherapy. By identifying specific characteristics of a patientβs tumour and immune system, biomarkers can help determine the most effective combination of nanomedicine and immunotherapy. Current tools like the Immunoscore and cancer immunogram are already being used in immunotherapy, and similar tools are needed for nano-immunotherapy to guide treatment decisions and optimise outcomes.
Challenges and Future Directions
The main challenge in nano-immunotherapy is understanding the complex interactions between nanoparticles and the immune system. This requires extensive research to develop effective treatments. Additionally, the manufacturing and regulatory hurdles associated with nanomedicines can slow their development and approval. Moreover, the effectiveness of nano-immunotherapy varies between patients, depending on factors such as cancer type and disease stage, emphasising the need for personalised approaches.
Nanomedicine and immunotherapy represent two of the most promising avenues in cancer treatment. While each has its limitations, their combination offers a powerful new approach. Nano-immunotherapy has the potential to enhance the effectiveness of cancer treatments, especially in resistant tumours, by making them more responsive to immune attack. However, realising this potential will require overcoming scientific and clinical challenges, including the development of predictive biomarkers and a deeper understanding of the interactions between nanomedicines and the immune system. As research continues, nano-immunotherapy could become a cornerstone of personalised cancer treatment, offering new hope to patients with difficult-to-treat cancers.
Nanomedicine and Tissue Engineering
The Role of Nanomedicine in Tissue Regeneration and Repair
As populations in developed countries continue to age, the prevalence of age-related diseases such as osteoarthritis, osteoporosis, and Parkinsonβs disease is on the rise. These conditions, along with factors like accidents and unhealthy habits such as smoking and chronic stress, often lead to tissue or organ dysfunction. In severe cases, tissue damage can even result in the loss of organ function. Traditionally, organ transplantation has been the go-to solution to restore health and prolong life. However, this approach is limited by a shortage of donor organs and the complications associated with lifelong immune suppression and other side effects.
Tissue Engineering:
A Promising Alternative
In recent years, tissue engineering has emerged as a promising alternative to organ transplantation. This interdisciplinary field aims to develop solutions that can replicate the functions of natural tissues, offering the potential to repair or replace damaged tissue. The ultimate goal of tissue engineering is to create constructs that can:
1. Mimic the characteristics of natural tissues.
2. Temporarily fill spaces left by damaged tissues while regeneration occurs.
3. Replace tissue functions temporarily.
4. Serve as a guide for new tissue growth.
Nanotechnology in Tissue Engineering
Nanotechnology plays a crucial role in advancing tissue engineering. By working with materials on a nanoscale (less than 100 nanometers), researchers are developing scaffolds that can temporarily replace the extracellular matrix (ECM) of damaged tissues. These scaffolds support the regeneration process by providing a structure that host cells can repopulate, eventually leading to the formation of new, natural tissue.
For these scaffolds to be effective, they must meet several critical criteria:
1. Mechanical Compatibility: The scaffold must match the mechanical properties of the tissue it is replacing to avoid a mismatch between the synthetic graft and the surrounding natural tissue. This ensures that the scaffold can withstand the physical forces in the body without causing further damage.
2. Biocompatibility: The materials used to construct the scaffold must be biocompatible, meaning they should not be toxic to the body or provoke an immune response. This is crucial for ensuring that the scaffold can support tissue regeneration without causing adverse effects.
3. Biodegradability: If the scaffold material is biodegradable, its degradation rate should align with the rate of tissue regeneration. This synchronization is essential for an optimal healing process, as it ensures that the scaffold gradually disappears as the new tissue forms.
4. Porosity: Adequate porosity is vital for allowing nutrients to reach the regenerating cells. The scaffold must facilitate the delivery of oxygen and other essential nutrients to support cell growth and tissue repair.
5. Nanotopography: The surface structure of the scaffold, or its nanotopography, should promote cell adhesion and proliferation. The right surface features can encourage cells to attach to the scaffold and multiply, accelerating the regeneration process.
Nanomedicine is at the forefront of innovations in tissue regeneration and repair, offering new hope for patients with damaged or dysfunctional tissues. By leveraging the unique properties of nanoscale materials, researchers are developing scaffolds that not only support the regeneration of natural tissues but also overcome many of the limitations associated with traditional treatments like organ transplantation. As this field continues to evolve, it holds the potential to transform how we approach tissue repair, ultimately improving outcomes for patients with a wide range of conditions.
Challenges in Nanomedicine for Tissue Engineering
Tissue engineering (TE) has the potential to revolutionize regenerative medicine, but several challenges remain, particularly the difficulty in replicating the natural properties of tissues using engineered materials. The materials currently used in TE often fall short in mimicking the complex mechanical and biological characteristics of natural tissues. This limitation has been a significant roadblock in the advancement of tissue engineering.
Nanotechnology: A Potential Solution
Nanotechnology offers promising solutions to this challenge by enabling the customization of nanoparticles to enhance both the mechanical and biological performance of engineered tissues. Recent developments have seen nanoparticles being increasingly utilized in tissue engineering to address these issues.
Future direction
The Future of Nanomedicine: Directions in Research and Development
Nanomedicine represents the frontier where science, technology, and medicine converge, utilizing nanoscale materials and devices to diagnose, treat, and prevent diseases, as well as to improve overall human health. The potential of nanomedicine is vast, encompassing everything from early disease detection to advanced regenerative medicine. As the field continues to evolve, several key areas are emerging as future directions for research and development.
Nanodiagnostics: Revolutionizing Early Disease Detection
One of the most promising areas of nanomedicine is nanodiagnostics, which involves the use of nanodevices to detect diseases at their earliest stages, often at the cellular and molecular levels. In vitro, nanotechnology enhances diagnostic techniques by improving the efficiency and accuracy of tests performed on human fluids or tissue samples. Nanodevices can conduct multiple analyses at a subcellular scale, offering a more detailed and precise understanding of a patient's health.
In vivo nanodiagnostics, on the other hand, focuses on devices that operate inside the human body. These nanodevices could potentially detect the early presence of diseases by identifying and quantifying toxic molecules, cancer cells, or other harmful entities. The ability to diagnose diseases at such an early stage would revolutionize healthcare, allowing for more effective treatments and better patient outcomes.
Regenerative Medicine: Repairing and Replacing Tissues and Organs
Regenerative medicine is another exciting frontier in nanomedicine, combining cell therapy and tissue engineering to repair, enhance, and maintain cells, tissues, and organs. Nanotechnology enables scientists to interact directly with cell components, manipulate cell proliferation and differentiation, and organize the production of extracellular matrices. These capabilities are essential for developing advanced regenerative therapies that could one day replace damaged tissues or even entire organs.
In the next 10 to 20 years, we may see the development of complex machines at the micrometer scale, composed of parts at the nanometer scale. These machines could include components such as nanomanipulators, molecular sorters, and incredibly durable surfaces made of flawless diamond. Such devices could be used to repair tissues at a molecular level, offering unprecedented precision in medical treatments.
The Role of Nanocomputers in Nanomedicine
As nanomechanical devices become more sophisticated, they will require equally advanced systems to control and operate them. This is where nanocomputers come into play. Nanocomputers could be tasked with activating, controlling, and deactivating nanomechanical devices, ensuring they function safely and effectively. These tiny computers would store and execute mission plans, process external signals, and communicate with other nanodevices or external monitoring systems. They would also possess the contextual knowledge necessary to adapt to their environment, further enhancing the safety and efficiency of nanomedicine applications.
Implications for Medicine and Beyond
The advancements in nanomedicine have enormous implications not only for medicine but also for fields like dentistry, where precision and control are crucial. As research and development continue, nanomedicine could lead to groundbreaking treatments that were once the stuff of science fiction. From repairing tissues at the molecular level to diagnosing diseases before they fully develop, the future of nanomedicine promises to transform healthcare in ways we are just beginning to imagine.
Conclusion
Nanomedicine is poised to change the landscape of modern medicine. With ongoing research and development, we are likely to see significant advancements in nanodiagnostics, regenerative medicine, and the integration of nanocomputers into medical applications. As these technologies mature, they will bring us closer to a future where diseases can be detected early, tissues can be repaired with precision, and health can be preserved and enhanced in ways previously thought impossible. The future of nanomedicine is not just about treating diseasesβitβs about redefining the possibilities of human health and longevity.