CITRIC ACID CYCLE - TCA CYCLE
Uncover the crucial pathway that powers life . The citric acid cycle (Krebs cycle), a vital metabolic process that converts food into energy, producing ATP, NADH, and FADH2. Discover how this ancient cellular process is essential for cellular respiration, energy production, and the survival of all living organisms.
CARBOHYDRATE METABOLISMBIOCHEMISTRY
CITRIC ACID CYCLE
☀The citric acid cycle (krebs cycle os tetracarboxylic acid TCA cycle) is the most important metabolic pathway for the energy supply to the body.
☀10% of 65-70% the ATP is synthesized on kreb cycle.
☀Citric acid, yield essential involves the oxidation of acetyl CoA to 102 and 160.
☀This ayde utilizes about 2/3nd off total O2 consumed by the body.
☀The citric acid cycle [TCA] proposed by Hans Adolf krebs in 1937, based on the studies of O2 consumption in pigeon breast muscles .
☀Citric acid is a common oxidative pathway carbonate for carbohydrates,fats and amino acid.
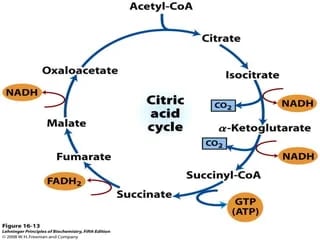
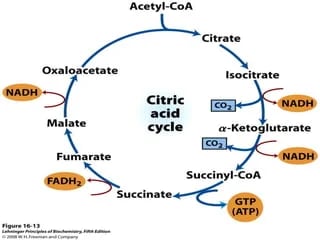
1.Formation of Citrate:
☀Acetyl-CoA and Oxaloacetate condense to form Citrate, catalyzed by Citrate Synthase.
The condensation of acetyl-CoA and oxaloacetate to form citrate is catalyzed by citrate synthase. This enzyme is crucial in regulating the pace of the TCA cycle, as it initiates the conversion of two-carbon acetyl units into a six-carbon molecule, citrate. Citrate serves not only as a substrate for subsequent reactions but also as an allosteric inhibitor of citrate synthase itself, thereby controlling its own production. This step links carbohydrate, fat, and protein metabolism since acetyl-CoA can be derived from various sources within the cell, ensuring flexibility in energy production pathways.
2.Isomerization of Citrate to Isocitrate:
☀Aconitase enzyme catalyzes the dehydration and subsequent hydration to form Isocitrate.
Following citrate formation, aconitase catalyzes the isomerization of citrate into isocitrate by removing a water molecule and subsequently adding it back in a different position. This reversible transformation is essential for preparing the substrate for the subsequent oxidative decarboxylation step. Aconitase's role highlights the dynamic nature of enzymatic control in metabolic pathways, ensuring efficient energy production while adapting to cellular demands and environmental conditions.
3.Formation of α-Ketoglutarate:
☀Isocitrate undergoes oxidative decarboxylation by Isocitrate Dehydrogenase (ICD), producing α-Ketoglutarate, along with NADH and CO2.
Isocitrate undergoes oxidative decarboxylation by isocitrate dehydrogenase (ICD), a pivotal step in the TCA cycle. This enzyme not only facilitates the release of a molecule of CO2 but also catalyzes the reduction of NAD+ to NADH. The resulting α-ketoglutarate is a critical intermediate that bridges carbohydrate metabolism with amino acid metabolism, as α-ketoglutarate serves as a precursor for glutamate and subsequently other amino acids. This step exemplifies the integrated nature of cellular metabolism, where molecules shuttle between pathways to meet diverse cellular needs.
4.Conversion of α-Ketoglutarate to Succinyl-CoA:
☀α-Ketoglutarate is oxidatively decarboxylated to Succinyl-CoA by α-Ketoglutarate Dehydrogenase Complex, generating NADH and CO2.
☀This step requires cofactors: TPP, Lipoamide, NAD+, FAD, and CoA.
α-Ketoglutarate is oxidatively decarboxylated to succinyl-CoA by the α-ketoglutarate dehydrogenase complex (KGDH), a multienzyme complex similar in structure and function to the pyruvate dehydrogenase complex. KGDH requires several cofactors—thiamine pyrophosphate (TPP), lipoamide, NAD+, FAD, and CoA—to facilitate the conversion. This step not only generates another molecule of NADH but also highlights the interconnectedness of metabolic pathways through shared enzymatic machinery. The production of succinyl-CoA provides the potential for substrate-level phosphorylation, contributing directly to ATP/GTP production.
5.Formation of Succinate:
☀Succinyl-CoA is converted to Succinate by Succinyl-CoA Synthetase, producing GTP/ATP via substrate-level phosphorylation.
Succinyl-CoA is converted to succinate by succinyl-CoA synthetase, which catalyzes the reversible phosphorylation of GDP or ADP to form GTP or ATP, respectively. This substrate-level phosphorylation represents a direct mechanism for energy transfer within the TCA cycle, demonstrating how chemical potential energy in the form of high-energy bonds is harnessed and utilized by the cell. The production of succinate also marks a key point where metabolic intermediates can be diverted towards biosynthetic pathways or further catabolized for energy.
6.Conversion of Succinate to Fumarate:
☀Succinate is oxidized to Fumarate by Succinate Dehydrogenase, generating FADH2.
Succinate undergoes oxidation by succinate dehydrogenase (SDH), a unique enzyme that is embedded in the inner mitochondrial membrane and serves a dual role in both the TCA cycle and the electron transport chain. As succinate is oxidized to fumarate, SDH transfers electrons to FAD, generating FADH2 in the process. This step underscores the intimate connection between the TCA cycle and oxidative phosphorylation, as electrons harvested from succinate oxidation are ultimately transferred to the electron transport chain for ATP synthesis.
7.Formation of Malate:
☀Fumarate is hydrated to Malate by Fumarase.
Fumarate is hydrated to form malate in a reaction catalyzed by fumarase. This hydration reaction adds a water molecule to fumarate, producing malate as a preparatory step for the final regeneration of oxaloacetate. The production of malate ensures the continuous flow of intermediates through the TCA cycle, maintaining metabolic homeostasis and meeting cellular energy demands under varying physiological conditions.
8.Regeneration of Oxaloacetate:
☀Malate is oxidized to Oxaloacetate by Malate Dehydrogenase, generating NADH.
☀Oxaloacetate then combines with another molecule of Acetyl-CoA to continue the cycle. The overall process results in the production of ATP/GTP, NADH, and FADH2, which are essential for cellular energy production and other metabolic processes.
Malate is oxidized to oxaloacetate by malate dehydrogenase, generating another molecule of NADH in the process. Oxaloacetate is then ready to combine with another molecule of acetyl-CoA to initiate a new cycle of the TCA cycle. This regeneration step completes the cycle, ensuring the continuous flux of carbon through the pathway and the sustained production of ATP, NADH, and FADH2 for oxidative phosphorylation.
Energetics of the Citric Acid Cycle:
☀During the oxidation of acetyl CoA via the Citric Acid Cycle, 4 reducing equivalents are produced.
Energy Production:
☀Oxidation of 3 NADH molecules through the electron transport chain typically yields enough energy to produce about 9 ATP molecules.
☀Additionally, 2 ATP molecules are directly synthesized through substrate-level phosphorylation in the cycle.
☀Therefore, a total of 12 ATP molecules are generated from one acetyl CoA molecule.
☀Enzymes of the Citric Acid Cycle:
- Aconitase
- Isocitrate dehydrogenase
- Alpha-ketoglutarate dehydrogenase
- Succinyl-CoA synthetase
- Succinate dehydrogenase
- Fumarase
- Malate dehydrogenase
Regulation of the Citric Acid Cycle:
1.Citrate Synthase:
- Inhibited by ATP, NADH, acetyl CoA, and succinyl CoA.
2.Isocitrate Dehydrogenase:
- Activated by ADP.
- Inhibited by ATP and NADH.
3.Alpha-Ketoglutarate Dehydrogenase:
- Activated by ADP.
- Inhibited by succinyl CoA and NADH.
4.Importance Of ADP
Adequate levels of ADP are crucial for maintaining the activity of the Citric Acid Cycle. Insufficient ADP levels can limit the oxidation of NADH and FADH2 through the electron transport chain steps.
☀The Citric Acid Cycle is a central pathway in cellular metabolism, generating ATP and reducing equivalents for oxidative phosphorylation. Proper regulation ensures efficient energy production and metabolic balance within the cell.
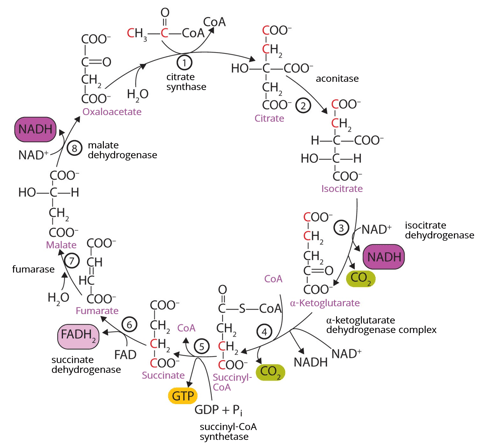
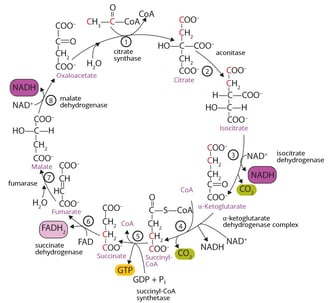